CLOCK
To evolve from apes 7 hrs
Homo Sapiens so far 10mins
Human history 30secs
1000 years 3secs
I year 3msec
Molecular Biology
of Life
Structures of life
Plant
Cellulose is a polysaccharide consisting of a linear chain of several hundred to many thousands of β(1→4) linked D-glucose units.[3][4] Cellulose is an important structural component of the primary cell wall of green plants, many forms of algae and the oomycetes. Some species of bacteria secrete it to form biofilms.[5] Cellulose is the most abundant organic polymer on Earth.[6] The cellulose content of cotton fibre is 90%, that of wood is 40–50%, and that of dried hemp is approximately 57%
Cells
Lipids for cell walls
Phospholipids PO4 head - ester link - hydrocarbon tail
Glycolipid Glucose C6H12O6 in a ester ring - ester link - hydrocarbon tail
Proteins
Proteins are large biomolecules and macromolecules that comprise one or more long chains of amino acid residues. Proteins perform a vast array of functions within organisms, including catalysing metabolic reactions, DNA replication, responding to stimuli, providing structure to cells and organisms, and transporting molecules from one location to another. Proteins differ from one another primarily in their sequence of amino acids, which is dictated by the nucleotide sequence of their genes, and which usually results in protein folding into a specific 3D structure that determines its activity.
Most proteins consist of linear polymers built from series of up to 20 L-α-amino acids. All proteinogenic amino acids have a common structure where an α-carbon is bonded to an amino group, a carboxyl group, and a variable side chain.
In biochemistry, denaturation is a process in which proteins or nucleic acids lose folded structure present in their native state due to various factors, including application of some external stress or compound, such as a strong acid or base, a concentrated inorganic salt, an organic solvent (e.g., alcohol or chloroform), agitation and radiation, or heat.
NASA expedition to asteroid Bennu which OSIRIS-REx delivered to Earth in 2023.
Discoveries include amino acids – 14 of the 20 that life on Earth uses to make proteins – and all five nucleobases that life on Earth uses to store and transmit genetic instructions in more complex terrestrial biomolecules, such as DNA and RNA, including how to arrange amino acids into proteins.
Keratinocytes are the primary type of cell found in the epidermis, the outermost layer of the skin. In humans, they constitute 90% of epidermal skin cells. Keratin is one of a family of structural fibrous proteins also known as scleroproteins. It is the key structural material making up scales, hair, nails, feathers, horns, claws, hooves, and the outer layer of skin in vertebrates.
In molecular biology, fibrous proteins or scleroproteins are one of the three main classifications of protein structure (alongside globular and membrane proteins).[1] Fibrous proteins are made up of elongated or fibrous polypeptide chains which form filamentous and sheet-like structures. This kind of protein can be distinguished from globular protein by its low solubility in water. In contrast, globular proteins are spherical and generally soluble in water, performing dynamic functions like enzymatic activity or transport. Such proteins serve protective and structural roles by forming connective tissue, tendons, bone matrices, and muscle fiber.
Soluble DNA and RNA for reproduction
The two DNA strands are known as polynucleotides as they are composed of simpler monomeric units called nucleotides.[2][3] Each nucleotide is composed of one of four nitrogen-containing nucleobases (cytosine [C], guanine [G], adenine [A] or thymine [T]), a sugar called deoxyribose, and a phosphate group. The nucleotides are joined to one another in a chain by covalent bonds (known as the phosphodiester linkage) between the sugar of one nucleotide and the phosphate of the next, resulting in an alternating sugar-phosphate backbone. The nitrogenous bases of the two separate polynucleotide strands are bound together, according to base pairing rules (A with T and C with G), with hydrogen bonds to make double-stranded DNA. The complementary nitrogenous bases are divided into two groups, the single-ringed pyrimidines and the double-ringed purines. In DNA, the pyrimidines are thymine and cytosine; the purines are adenine and guanine.
Di and tri phosphates for energy management
ATP & ADT. When consumed in a metabolic process, ATP converts either to adenosine diphosphate (ADP) or to adenosine monophosphate (AMP)
Neurons
Like all animal cells, the cell body of every neuron is enclosed by a plasma membrane, a bilayer of lipid molecules with many types of protein structures embedded in it.[12] A lipid bilayer is a powerful electrical insulator, but in neurons, many of the protein structures embedded in the membrane are electrically active. These include ion channels that permit electrically charged ions to flow across the membrane and ion pumps that chemically transport ions from one side of the membrane to the other. Most ion channels are permeable only to specific types of ions. Some ion channels are voltage gated, meaning that they can be switched between open and closed states by altering the voltage difference across the membrane. Others are chemically gated, meaning that they can be switched between open and closed states by interactions with chemicals that diffuse through the extracellular fluid. The ion materials include sodium, potassium, chloride, and calcium. The interactions between ion channels and ion pumps produce a voltage difference across the membrane, typically a bit less than 1/10 of a volt at baseline. This voltage has two functions: first, it provides a power source for an assortment of voltage-dependent protein machinery that is embedded in the membrane; second, it provides a basis for electrical signal transmission between different parts of the membrane.
Hemoglobin
Hemoglobin has a quaternary structure characteristic of many multi-subunit globular proteins.[43] Most of the amino acids in hemoglobin form alpha helices, and these helices are connected by short non-helical segments. Hydrogen bonds stabilize the helical sections inside this protein, causing attractions within the molecule, which then causes each polypeptide chain to fold into a specific shape.[44] Hemoglobin's quaternary structure comes from its four subunits in roughly a tetrahedral arrangement.[43]
In most vertebrates, the hemoglobin molecule is an assembly of four globular protein subunits. Each subunit is composed of a protein chain tightly associated with a non-protein prosthetic heme group. Each protein chain arranges into a set of alpha-helix structural segments connected together in a globin fold arrangement. Such a name is given because this arrangement is the same folding motif used in other heme/globin proteins such as myoglobin.[45][46] This folding pattern contains a pocket that strongly binds the heme group.[citation needed]
A heme group consists of an iron (Fe) ion held in a heterocyclic ring, known as a porphyrin. This porphyrin ring consists of four pyrrole molecules cyclically linked together (by methine bridges) with the iron ion bound in the center.[47] The iron ion, which is the site of oxygen binding, coordinates with the four nitrogen atoms in the center of the ring, which all lie in one plane. The heme is bound strongly (covalently) to the globular protein via the N atoms of the imidazole ring of F8 histidine residue (also known as the proximal histidine) below the porphyrin ring. A sixth position can reversibly bind oxygen by a coordinate covalent bond,[48] completing the octahedral group of six ligands. This reversible bonding with oxygen is why hemoglobin is so useful for transporting oxygen around the body.[49] Oxygen binds in an "end-on bent" geometry where one oxygen atom binds to Fe and the other protrudes at an angle. When oxygen is not bound, a very weakly bonded water molecule fills the site, forming a distorted octahedron. Blood in Arthropods (insects & shellfish) have Cu in place of Fe.
Hemoglobin has a remarkable resemblance to Chlorophyl, which has Magnesium in the same location as Fe in Hemoglobin. Chlorophyl is the absorbs blue and red light for photosynthesis, hence leaves are green. It sits in a cell wall in the form of dimers P680 and P700.
P680 receives excitation energy either by directly absorbing a photon of suitable frequency or indirectly from other chlorophylls within photosystem II, thereby exciting an electron to a higher energy level. The resulting P680 with a loosened electron is designated as P680*, which is a strong reducing agent.
Following excitation, the loosened electron of P680* is taken up by the primary electron acceptor, a pheophytin molecule located within photosystem II near P680. During this transfer, P680* is ionized and oxidized, producing cationic P680+.
P680+ is the strongest biological oxidizing agent known, with an estimated redox potential of ~1.3 V.[3] This makes it possible to oxidize water during oxygenic photosynthesis. P680+ recovers its lost electron by oxidizing water via the oxygen-evolving complex, which regenerates P680.
It seems a small leap that a strong oxidizing agent might mutate from Mg to Cu and Fe and evolve oxygen transport properties.
Life Elsewhere
Species requires a huge variety of different structures. Life on earth uses water soluble carbon polymers linked to phosphorous salts as cell walls, DNA, RNA, energy transfer compounds ATP and ADT, enzymes.... the myriad of molecules required for life.
Carbon forms the backbone of a huge variety of small molecules and polymers. Carbon based polymers can have a huge range of physical properties, but are generally hydrophobic. For molecular mobility at room temperature, these molecules need to be dissolved in a solvent. Water is a naturally occurring liquid from 0C to 100C, and is widespread in the universe. Carbon polymers need to be linked to an ionizable atom to make it hydrophilic and water soluble. Phosphorous has one valence electron more than carbon, so it can support a single negative charged polar head in a C-P bond. The variety of hydrophobic and hydrophilic properties enable different structures and control of molecular shapes.
In a planet with water, carbon/phosphorous molecules have the range of properties required. We know that life of earth has a huge variety of forms from humans to octopus all showing intelligence.
Colder planets could have other solvents such as nitrogen, methane. These are hydrophobic and would require a very different molecular strategy to enable structural variety.
Best guess - other life will be on a water planet based on carbon chemistry. It will probably not look like any life on earth.
Function evolution
Metabolism starts with Photosynthesis in bacteria and plants the uses light energy to combine carbon dioxide and water to fix carbon producing the full range of biomolecules and oxygen, catalyzed by enzymes in the Calvin Cycle. Plants expanded their metabolism to grow cellulose shells for huge multicellular structures, and added more efficient energy generation from chloroplasts to become prolific oxygen generators.
The oxygen produced by photosynthesis is then used in aerobic respiration to generate energy stored by converting a diphosphate (ADP) to a triphosphate (ATP) and carbon dioxide, catalyzed by enzymes in the Krebs Cycle. Fungi were the first "digesters" that used the Krebs Cycle Fungi also fabricate new biomolecules to grow using RNA bound to amino acids to build a peptide chain.
Animals evolve ways convert the biomolecules in plants and animals in food to glucose using their new specialized organs. Animals expanded their metabolism to include oxygen supply using blood, in using energy to trigger molecular reconfiguration in muscles, and electrical transfer in nerves and neurons, and the growth of biomolecules. These reactions combine monosaccharides to form polysaccharides, fatty acids to form triglycerides, amino acids to form proteins, and nucleotides to form nucleic acids. These processes require energy in the form of ATP molecules generated by catabolic reactions. Anabolic reactions, also called biosynthesis reactions, create new molecules that form new cells and tissues, and revitalize organs.
The first animals were all cold blooded. The evolution of warm blood enables higher metabolic rates with enhanced unloading of oxygen by hemoglobin. This relationship is helpful as metabolically-active peripheral tissues such as exercising skeletal muscle which often display supra-normal temperatures. Because of this increased temperature, oxygen unloading by hemoglobin is enhanced in these metabolically-active tissues, thus improving oxygen transport to areas which require it most.
https://courses.lumenlearning.com/suny-ap2/chapter/energy-and-heat-balance/
https://portlandpress.com/essaysbiochem/article/64/4/607/226177/Metabolism
Transport
The animals use blood to concentrate and deliver oxygen. Copper blood carries 1/4 of the oxygen as iron blood (haemoglobin), but works better at low temperature and may have less tendency to clot.
Copper blood first appeared in mollusks with open circulation throughout their body cavity.
Closed circulation with arteries and veins evolved to better deliver oxygen and nutrients to key organs in vertebrates and cephalopods like octopus.
The vertebrates then evolved with more efficient iron based blood.
Finally amphibians evolved lungs that enabled complex air breathing life.
Structure
Bacteria started with dual lipid based cell walls.
Plants added a cellulose superstructure that enabled large trees.
Exoskeletons appeared with the first mollusks that provided physical protection and a structure for load bearing and locomotion. The big limitation was that the skeleton does not grow, it must be shed and regrow for the animal to grow larger.
The evolution of vertebrates with an internal support structure started with fish and continues today. The basic bone architecture has been retained for hundreds of millions of years. The internal structure allows growth and training changes.
Reproduction
It starts with cell division in bacteria. Plants use seeds usually spread annually.
The first animals reproduced through large numbers of fertilized eggs, that were left to fend for themselves. Eggs provide a limited energy supply and when it is used the fetus must be self supporting.
An improved survival strategy appeared where eggs are protected by parents (octopus) before birth. Warm blooded animals had to keep the eggs warm as well (birds). After birth, the infants are often fed by parents until self-supporting.
Another survival strategy that did not require fully developed digestive systems appeared in monotremes. After eggs are hatched, the infants are supported by mothers milk
One way to improve survival appeared when eggs were allowed to develop protected inside the mothers body, for example in sharks.
The final evolution occurred in placental mammals, where the fetus develops with unlimited energy and time, with energy supplied by the placenta inside the mother, and then is supported after birth by mothers milk.
Brain capability
-
Humans and large marine mammals have 10B's of sensory neurons with unique capabilities
-
Most primates, smaller marine mammals, very large herbivores (elephants), smartest birds (rooks, macaw's) have 1B's and are accepted as really "smart" in that they can identify themselves in mirrors and use tools. .
-
The majority of mammals both herbivore and predators (domestic dogs rank highest!), birds and octopus have 0.1B's capable of significant strategic thinking. Probably groups with the smartest dinosaurs such as the raptors.
-
Small mammals and many birds have 0.01B's.
-
Insects and fish check in at 0.001B's.
Large body size seems to correlate to high neuron count. In humans, self partner selection may explain the particularly high neuron count. In domestic dogs, breed selection may explain the high counts.
On the average the egg laying birds have lower neuron counts than mammals, but the highest birds match primates. Along with octopus it would suggest that placental birth may have helped but is not essential for developing intelligence.
The table to the right gives information on the number of neurons estimated to be in the sensory-associative structure: the cerebral cortex (aka pallium) for mammals, the dorsal ventricular ridge ("DVR" or "hypopallium") of the pallium for birds, and the corpora pedunculata ("mushroom bodies") for insects.
https://en.wikipedia.org/wiki/List_of_animals_by_number_of_neurons
It appears that the recipe for intelligence is a creature with locomotion, a closed circulation oxygen deliver system for key organs, and a complex gene structure that can be locally reorganized.
The most intelligent species seem to track the brain to body weight ratio, but requires a long time to reach maturation.
Primates are large tree dwellers which protects them from predation.
Elephants have great size which protects them.
Octopuses have remarkable camouflage for protection.
Cetaceans (whales & dolphins) are the largest water dwellers.
Crows show remarkable adaptability and the ability to problem solve.
Small mammals, such as rodents, were prey for many larger species. Survival requires mobility with little time for maturation. This would block evolution of greater intelligence.
Cats actually have 90.2% of the DNA in common with us! You read that right! Cats are genetically surprisingly closer to us than dogs, who share about 84% of the genes with us (Pontius et al, 2007).
Match to Human DNA
Plants (18%) 1.5 B years for 82% change 18 My/%
Insects (44%) 500M years 66% 9 My/%
Birds (80%) 200 M years for 20% 10 My/%
Dogs 84%
Cats (90.2%)
Rodents (93%) 80 M years for 7% 11 My/% Apes (99%) 7 M years for 1% 7 My/%
Humans (100%)
It took 1.5 By for plants to evolve to Dinosaurs (80% human DNA), who then lasted for 200My. Insects have 60% human DNA. It took 60My for Shrews (93% human DNA) to evolve to Apes (99.5% human DNA). It took 7M years for Apes to evolve to Humans. The DNA differences represent a "round trip", so the mutation rate is around 20M years for 1% DNA change in gene location.
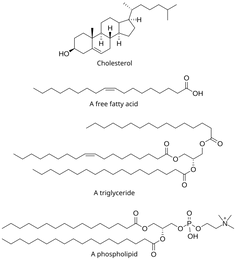
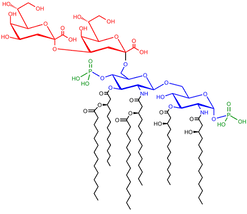

Myglobin
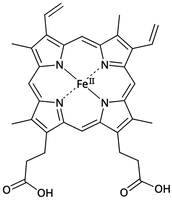


